Group leader: Dr. Esther Hulleman
(Combinational) Drug screens:
To identify compounds that can be incorporated into new treatment schemes, we perform (semi) high-throughput drug screens on primary cell cultures, either as monotherapy or in combination with other (epigenetic) drugs or gamma-irradiation. Radiotherapy is an important component of the current treatment schedules for most types of high-grade brain tumor in children, and – as such – should be considered when screening for novel agents. Drug screen libraries consist of both ‘classical chemotherapeutics’, and targeted agents: epigenetic-, kinase- or pathway inhibitors, depending on the methylation- and gene expression data of the primary tumor. In addition, we use gene expression profiling and protein phosphorylation arrays that enable us to identify signal transduction routes that are essential for the growth of tumor cells, as well as pathways that are involved in escape mechanisms leading to drug resistance. This allows a rational design of combinational therapies, in which the drugs act with an additive or synergistic effect. Preferably, we test compounds that pass the blood-brain barrier (BBB) and have been proven safe in children and approved for the European market, to enable a quick translation to the clinic.
Tumor Biology:
Besides the abovementioned drug screens, we aim to develop novel therapies based on the biology/immunology of pediatric brain tumor (sub)types, with a special focus on crosstalk with healthy brain- or immune cells and therapy resistance. In recent years, an increasing number of publications has shown that brain tumors closely interact with neighboring healthy cells and infiltrating immune cells to support their growth and survival.
To determine the feasibility of immunotherapy in, we investigate the role of the tumor immune microenvironment (TIME) in various pediatric brain tumor (sub)types and explore the possibility to alter their immunophenotype using co-cultures and immunocompetent in vivo models, such as the murine syngeneic grafts. Therefore, we map changes in TIME upon treatment with immunomodulating agents and perform synthetic lethality screens using CRISPR (clustered regularly interspaced short palindromic repeats)/Cas9 screening methods. This technique can also be applied to identify pathways involved in therapy resistance, both in in vitro and in in vivo experiments where we can consider the role of the tumor microenvironment, BBB integrity and tumor heterogeneity. Again, we use different approaches to identify therapeutic targets: unbiased screens in which drug and/or irradiation treatment is combined with (inducible) CRISPR/Cas9 knock-outs, or a more rationalized approach in which we compare the gene expression profiles and pathway activation before-, and after treatment in resected patient material (provided that a second biopsy or debulking of the tumor is performed upon recurrence), or by exposing cell cultures to low drug concentrations for an extended period of time.
Drug delivery:
The minimal progress in the treatment of pediatric brain tumors in the past decades may be attributed to a limited drug distribution in the brain. Most chemotherapeutics – and small molecules in particular – are good substrates of drug efflux transporters, as they have historically been selected for their inability to pass the BBB to minimalize neurological damage and treatment related side effects. However, good brain penetration and subsequent drug accumulation will be essential for the treatment of (pediatric) brain tumors. Thus, novel treatment strategies (both invasive- and non-invasive) need to be developed, such as convection enhanced-, intra-arterial-, or intranasal delivery, sonoporation, implanted therapies, the use of nanocarriers, chemical modification of parent drugs with vectors that help crossing the BBB, or co-administration of chemotherapeutic agents with compounds that open up the BBB.
Novel research models:
Since the focus or our laboratory experiments is on translational projects, we need robust in vitro and in vivo models that mimic the molecular and histological phenotype of the tumors, to evaluate novel treatment modalities. Currently, such models are scarce, and most exhibit relatively slow tumor growth, making them unsuitable to test new therapies. Therefore, we routinely establish novel patient-derived xenograft (PDX) models for various forms of high-grade pediatric brain tumors from primary patient material. These models are extensively characterized on different levels (gene-expression profiles, genomic characteristics, and histology) and compared to the original tumor, to ensure their reliability. Besides drug testing, the PDX models can be used to study the blood-brain barrier, tumor biology, tumor-stroma interactions, or novel neurosurgical- and diagnostic techniques.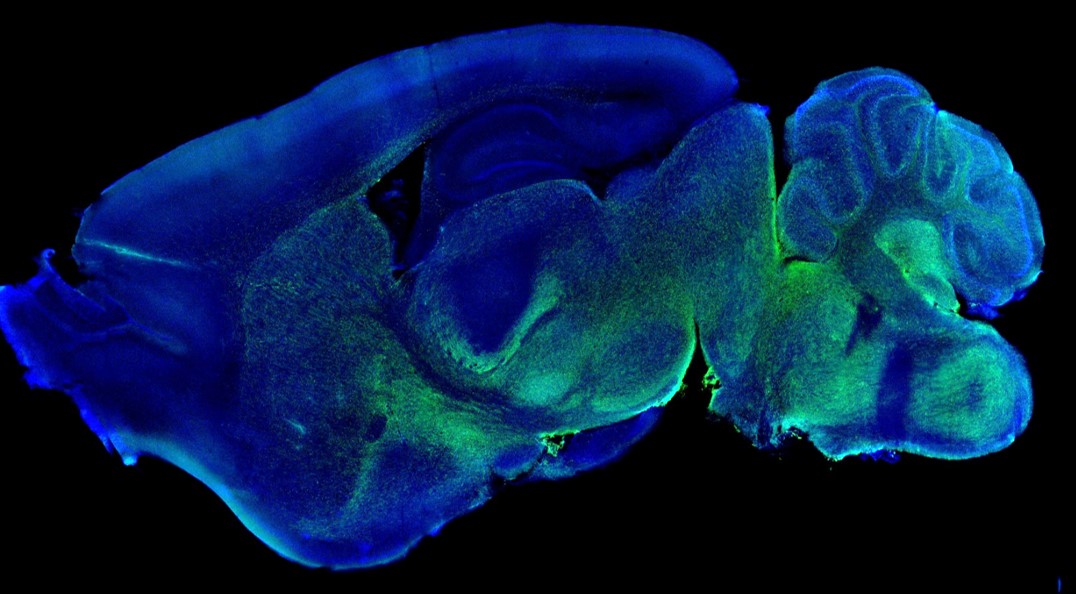
- DIPG/DMG Collaborative: "Assessing the potential of the HDAC inhibitor givinostat ± radiotherapy for the treatment of diffuse midline glioma.” (2023). On behalf of the following supportive organizations: The Cure Starts Now Foundation, Brooke Healey Foundation, The Cure Starts Now Australia, Jeffrey Tomas Hayden Foundation, Melina Michelle Edenfield Foundation, Reflections of Grace Foundation, Yuvaan Tiwari Foundation Cure Brain Cancer Foundation, Aubreigh’s Army Foundation 328, Run DIPG, The Cure Starts Now Canada, Aidan’s Avengers, Musella Foundation, Laurie’s Love Foundation, Love4Lucas Foundation, Whitley’s Wishes, Anna’s Bake Sale Foundation, Love Chloe Foundation, Lauren’s Fight for Cure, The Isabella and Marcus Foundation, Ryan’s Hope, Robert Connor Dawes Foundation, The Gold Hope Project, Abby’s Corner Foundation, The DIPG/DMG Collaborative and Snapgrant.com
- KWF (Dutch Cancer Foundation) "Towards combination therapy for diffuse midline glioma: exposing the immunomodulatory effects of the dopamine receptor D2 antagonist ONC201". (2023)
- KiKa (Children Cancer-free) Foundation “In vivo functional genetic screening for the identification of synthetically lethal therapeutic targets in atypical teratoid/rhabdoid tumors”. (2022)
- Koppie Au “microglia in brain tumors” (Dennis Metselaar) (2022)
- STOPhersentumoren.nl “a novel therapeutic strategy for the treatment of PF-A pediatric ependymoma”. (2021)
- KiKa (Children Cancer-free) Foundation “DNA methylation profiling of pediatric brain tumors using liquid biopsies”. (2021)
- Koppie Au “preclinical evaluation of gemcitabine for the treatment of SHH medulloblastoma”. (2021)
- Semmy Foundation “Identification of therapeutic targets for diffuse midline glioma. (2019)
- KiKa (Children Cancer-free) Foundation “Enabling preclinical immunotherapeutic studies for pediatric diffuse midline glioma”. (2019)
- Egbers Foundation “Characterization of migrating tumor cells in pediatric high-grade glioma. (2018)
Honors and Awards:
- 2018: Tom Voute young investigator award (Hans Meel).
- 2008: AACR-Pezcoller Foundation Scholar-in-training Award
- 2001-2003: Marie-Curie Individual Fellowship
Microglia in paediatric brain tumours: the missing link to successful immunotherapy. Du Chatinier A, Querol Velilla I, Meel MH, Hoving EW, Hulleman E*, Metselaar DS. (2023). Cell Rep Med 4(11),101246.
Oncolytic DNX-2401 Virus for Pediatric Diffuse Intrinsic Pontine Glioma. Gállego Pérez-Larraya J, Garcia-Moure M, Labiano S, et al. (2022). N Engl J Med, 386(26): 2471-2481.
Generation of immunocompetent syngeneic allograft mouse models for pediatric diffuse midline glioma. du Chatinier A, Meel MH, Das AI, Metselaar DS, Waranecki P, Bugiani M, Breur M, Simonds EF, Lu ED, Weiss WA, Garcia Vallejo JJ, Hoving EW, Phoenix TN, Hulleman E. (2022). Neurooncol Adv, 4(1): vdac079.
AURKA and PLK1 inhibition selectively and synergistically block cell cycle progression in diffuse midline glioma. Metselaar DS, du Chatinier A, Meel MH, Ter Huizen G, Waranecki P, Goulding JR, Bugiani M, Koster J, Kaspers GJL, Hulleman E. (2022) iScience 25(6):104398.
Overview of current drug delivery methods across the blood-brain barrier for the treatment of primary brain tumors. Haumann R, Carvalho Videira J, Kaspers GJL, van Vuurden DG, Hulleman E. (2020). CNS Drugs, 34(11):1121-1131.
Combined treatment with CBP and BET inhibitors reverses inadvertent activation of detrimental super enhancer programs in DIPG cells. Wiese M, Hamdan F, Kubiak K, Diederichs C, Gielen G, Nussbaumer G, Carcaboso A, Hulleman E, Johnsen S, Kramm C. (2020). Cell Death Dis 11(8), 673.
Combined therapy of AXL and HDAC inhibition reverses mesenchymal transition in diffuse intrinsic pontine glioma. Meel MH, de Gooijer MC, Metselaar DS, Sewing ACP, Zwaan K, Waranecki P, Breur M, Buil LCM, Lagerweij T, Wedekind LE, Twisk JWR, Koster J, Hashizume R, Raabe EH, Montero Carcaboso Á, Bugiani M, Phoenix TN, van Tellingen O, van Vuurden DG, Kaspers GJL, Hulleman E. (2020). Clin Cancer Res, 26(13), 3319-3332.
Development of transient radioresistance during fractionated irradiation in vitro. Van den Berg J, Castricum KCM, Meel MH, Goedegebuure RSA, Lagerwaard FJ, Slotman BJ, Hulleman E*, Thijssen VLJL. (2020). Radiother Oncol 148, 107-114.
Immunotherapy in pediatric brain tumors: considerations, challenges and future directions. Kruithof ES, van der Lugt J, Hulleman E. (2019). J Mol Clin Med 2(3), 79-91.
Celastrol-induced degradation of FANCD2 sensitizes pediatric high-grade gliomas to the DNA-crosslinking agent carboplatin. Metselaar DS, Meel MH, Benedict B, Waranecki P, Koster J, Kaspers GJL, Hulleman E. (2019). Ebiomedicine 50, 81-92.
Signaling pathways and mesenchymal transition in pediatric high-grade glioma. Meel MH, Schaper SA, Kaspers GJL, Hulleman E. (2018). Cell Mol Life Sci, 75, 871-887. PubMed PMID: 29164272
Preclinical evaluation of convection-enhanced delivery with liposomal doxorubicin to treat pediatric diffuse intrinsic pontine glioma and thalamic high-grade glioma. Sewing ACP, Lagerweij T, van Vuurden DG, Meel MH, Veringa SJE, Montero-Carcaboso A, Gaillard PJ, Vandertop WP, Wesseling P, Noske DP, Kaspers GJL, Hulleman E. (2017). J Neurosurg Pediatr 17, 1-13. PubMed PMID: 28291423
Bevacizumab targeting diffuse intrinsic pontine glioma: results of 89Zr-Bevacizumab PET imaging in brain tumor models. Jansen MHA, Lagerweij T, Sewing ACP, Vugts DJ, van Vuurden DG, Molthoff CFM, Caretti V, Veringa SJE, Petersen N, Montero-Carcaboso A, Noske DP, Vandertop WP, Wesseling P, van Dongen GAMS, Kaspers GJL, Hulleman E. (2016). Mol Cancer Ther 15, 2166-2174. PubMed PMID: 27325687
Functionally defined therapeutic targets in diffuse intrinsic pontine glioma. Grasso CS, Tang Y, Truffaux N, Berlow NE, Liu L, Debily MA, Quist MJ, Davis LE, Huang EC, Woo PJ, Ponnuswami A, Chen s, Johung TB, Sun w, Kogiso M, Du Y, Qi L, Huang Y, Hutt-Cabezas M, Warren KE, Le Dret L, Meltzer PS, Mao H, Quezado M, van Vuurden DG, Abraham J, Fouladi M, Svalina MN, Wang N, Hawkins C, Nazarian J, Alonso MM, Raabe E, Hulleman E, Spellman PT, Li XN, Keller C, Pal R, Grill J, Monje M. (2015) Nat Med 21, 555-559. PubMed PMID: 25939062